Genomic abnormalities are one of the main challenges that scientists working on induced pluripotent stem cells have to overcome. They range from point mutations to large copy number changes, including full chromosome aneuploidy. If they occur, they have the potential to create a number of issues including invalidating research, impacting differentiation or creating health issues in patients. In short, iPSC scientists cannot afford to ignore them.
However, do we know the actual impact of finding abnormalities in hPSCs? Will they have the same consequences regardless of the type of culture and differentiated material? What Keller and Spits stated is still partially true: it is fair to say that little is known about how these abnormalities will impact the clinical translation of hPSC and particularly their potential to prime cells for oncogenic transformation (Keller & Spits, 2021). However, our understanding has been evolving rapidly since then, and some robust research work has started to emerge providing evidence of the impact of some abnormalities on hPSC cultures.
In this blog article, we will provide a summary of the most commonly found genomic defects in hPSCs and their known consequences. We will also discuss the strategies scientists can implement when faced with a genomic abnormality in the cell line they are working on.
Recent research has identified a broader range of genetic alterations, including copy number variations (CNVs) and single nucleotide variations (SNVs). Specific amplifications on chromosomes 1, 12, 17 and 20 are frequently observed, with gains in the 20q11.21 region being particularly common in hPSC lines (Figure 1) (Maitra et al., 2005; Amps et al., 2011).
Many of these abnormalities exist in a mosaic state in culture, which complicates their detection with conventional karyotyping methods. The figure below shows the most common abnormalities found in a sample of hPSC using digital PCR, a highly-sensitive technology*.
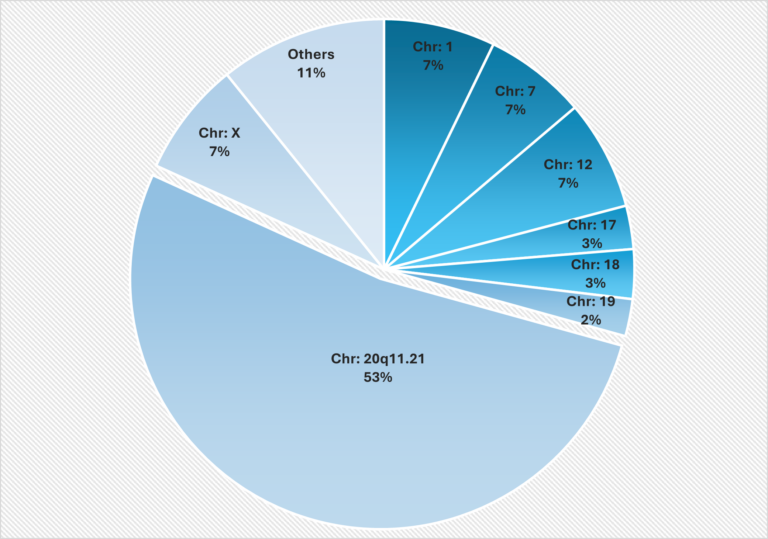
Figure 1: Localization of the most frequent genomic abnormalities observed in Stem Genomics testing using digital PCR
This figure illustrates the distribution of genetic abnormalities in human pluripotent stem cells (hPSCs) across different chromosomes. The most affected region was 20q11.21, followed by chromosomes 12, 7, 1 and X, which showed similar patterns. The data, derived from 2,124 abnormal samples, identified 2,374 chromosomal abnormalities, as some samples had multiple alterations. This proprietary data was provided by Stem Genomics. (Extract from the white paper “Ensuring genetic integrity in Pluripotent Stem Cells: challenges and solutions”. Author: Dr. Reda ZENAGUI)
* Digital PCR is a highly-sensitive technology that makes it possible to capture abnormalities in a mosaic state in cultures. Read more about the relevance of using digital PCR technology to detect abnormalities in PSC cultures.
1.1. CNVs: what we know about the consequences of the observed abnormalities
Chromosomes | Known consequences of the observed abnormalities | References |
20q | The 20q abnormality represents more than 20% of the abnormalities found in stem cells. However, the gains found in this region can be as small as 5 Mb and are often not detectable by a traditional method such as karyotyping. Gains in the 20q11.21 region often involve the BCL2L1 gene, which encodes 2 protein isoforms: Bcl-xL (anti-apoptotic) and Bcl-xS (pro-apoptotic). Abnormal cells show an overexpression of Bcl-xL isoform that confers a growth advantage to the culture. Neuroectodermal differentiation of 20q11.21 abnormal cells is strongly impaired. BCL2L1 gene overexpression is also described in various human cancers such as colorectal cancer. Gains in the 20q region are often below the resolution of karyotyping in the event of an interstitial duplication. | (Avery et al., 2013; Beroukhim et al., 2010; Diep et al., 2006; Kim et al., 2023; Markouli et al., 2019; Molina-Ruiz et al., 2022; Nguyen et al., 2014)
Standards for human stem cell use in Research, June 2023, ISSCR (https:/www.isscr.org/standards-document, accessed on 23/08/29) |
12p | Chromosome 12 trisomy causes an increase in the proliferation rate and DNA replication due to an overexpression of the pluripotency gene NANOG (12p13.31). This duplication can be associated with a decrease in spontaneous differentiation and apoptosis due to the duplication of the 12p11.2–12 region that contains KRAS and SOX5 genes. Gains in the 12p region promote genetic instability and tumorigenicity. These abnormalities are also found in testicular germ cell tumors and embryonal carcinoma. Differentiation may be affected. | (Atkin & Baker, 1982; Baker et al., 2007; Ben-David et al., 2014; Draper et al., 2004; Molina-Ruiz et al., 2022) |
1q | The 1q region contains the p53 regulator gene MDM4 which confers a selective advantage when duplicated. This gain has been described in many cancers, underlining the need for its detection in cells intended for cell therapy. Differentiation may be affected. | (Keller & Spits, 2021) |
Xp | The Xp anomaly is often seen alongside a chromosome 12 or 17 abnormality. A gain of the X chromosome affects the X-inactivation process. A study showed that X inactivation is effective after differentiation, but another study showed that X inactivation failed even after differentiation. Moreover, cell signaling molecules and receptors involved in cell cycle progression are on the X chromosome. | (Baker et al., 2007) |
17p | The short arm of chromosome 17 is the region of the well-known tumor suppressor gene TP53. Deletions of this region are associated with an increase in proliferation that confers a growth advantage, even in restrictive conditions (basic media, for example). A higher expression of the pluripotency gene POU5F1/OCT4 is observed in the del17p cells and confers resistance to the differentiation process. Mutant embryonic bodies (EB) show irregular projections and a loss of attachment efficiency. | (Amir et al., 2017; Garitaonandia et al., 2015)
|
18q | Loss of 18q in cell lines affects their differentiation efficiency and quality in Retinal Pigment Epithelium (RPE). Some of these cell lines also express a high level of undifferentiated markers. | (Lei et al., 2025) |
Source: Stem Genomics
1.2. What about SNVs?
Advanced testing technologies such as Whole Genome or Next Generation Sequencing have assisted in the discovery of new genomic abnormalities. These abnormalities are primarily chromosomal defects and point mutations (SNVs) that can spontaneously appear in hPSC lines in prolonged cultures.
Genes such as BCL2L1 and TP53 can trigger the proliferation of resistant cells, which in turn leads to the clonal expansion of genetically abnormal cells, disrupting differentiation capacities, gradually taking over the culture and increasing the risk of cancer after transplants in patients (Merkle et al., 2017, 2022), (Avery et al., 2013). Therefore, these mutations raise serious concerns about the potential for tumorigenesis when these genetically modified cells are used in therapy and require close monitoring (Ben-David et al., 2014).
Another well-documented SNV is the BCOR mutation. In their paper, (Rouhani et al., (2022) revealed strong selection pressure for BCOR mutations in F-hiPSCs and B-hiPSCs and provided evidence that they arise in vitro. Directed differentiation of hiPSCs and RNA sequencing showed that BCOR mutations have functional consequences. Their work strongly suggests that detailed nucleotide-resolution characterization is essential before using hiPSCs.
2. Strategies scientists can apply when faced with genomic abnormalities
- Do nothing: this is the strategy followed by scientists who know for a fact, based on published and credible research, that the identified variant will not affect the proper development of the type of differentiation protocol they work on or will not transform into pathogenic disease later on in patients. Another possible scenario is the existence of this mutation in the donor germline. Significant genetic diversity already exists within the population, with copy number variations being reported across multiple different tissue types, and it has been reported that individuals can carry a significant mutational burden in cancer-related genes without them becoming pathogenic (Keller & Spits, 2021). It is therefore very relevant to compare somatic mutations in the derived product with the germline genetic profile to draw final conclusions on the actual significance of the identified abnormalities.
- Test again in a few passages: this strategy is applied when abnormalities are suspected (commonly known as “trends”). As some damaged cells can be eliminated over time through apoptosis, it is therefore advisable to test again 5 to 10 passages later (Draper et al., 2004) to look at the evolution of the abnormalities identified.
- Go back to an earlier passage, stored in your working or master cell bank: Before starting the culture over again using material from an earlier passage, it is of course recommended to test for the identified abnormalities. This should be done with a sensitive technology such as digital PCR or NGS on an earlier passage, as sometimes the problem can re-emerge if the variant was below the level of detection. This is particularly relevant if the acquired cell line was tested for genomic abnormalities with low sensitivity techniques such as G-Banding karyotyping.
- Attempt to rescue your wild-type cells from your tested sample: for many people who are years into their work, or if their cell lines are rare and precious, throwing your cell line away is not feasible or cost-effective. It is therefore advisable to use single cell cloning to separate and identify normal cells (the wild type) present in a population and continue the culture by isolating them from the mutated cells. Read more about this technique.
Amir, H., Touboul, T., Sabatini, K., Chhabra, D., Garitaonandia, I., Loring, J. F., Morey, R., & Laurent, L. C. (2017). Spontaneous Single-Copy Loss of TP53 in Human Embryonic Stem Cells Markedly Increases Cell Proliferation and Survival. Stem Cells, 35(4), 872–885. https://doi.org/10.1002/stem.2550
Atkin, N. B., & Baker, MarionC. (1982). SPECIFIC CHROMOSOME CHANGE, i(12p), IN TESTICULAR TUMOURS? The Lancet, 320(8311), 1349. https://doi.org/10.1016/S0140-6736(82)91557-4
Avery, S., Hirst, A. J., Baker, D., Lim, C. Y., Alagaratnam, S., Skotheim, R. I., Lothe, R. A., Pera, M. F., Colman, A., Robson, P., Andrews, P. W., & Knowles, B. B. (2013). BCL-XL mediates the strong selective advantage of a 20q11.21 amplification commonly found in human embryonic stem cell cultures. Stem Cell Reports, 1(5), 379–386. https://doi.org/10.1016/j.stemcr.2013.10.005
Baker, D. E. C., Harrison, N. J., Maltby, E., Smith, K., Moore, H. D., Shaw, P. J., Heath, P. R., Holden, H., & Andrews, P. W. (2007). Adaptation to culture of human embryonic stem cells and oncogenesis in vivo. In Nature Biotechnology (Vol. 25, Issue 2, pp. 207–215). https://doi.org/10.1038/nbt1285
Ben-David, U., Arad, G., Weissbein, U., Mandefro, B., Maimon, A., Golan-Lev, T., Narwani, K., Clark, A. T., Andrews, P. W., Benvenisty, N., & Carlos Biancotti, J. (2014). Aneuploidy induces profound changes in gene expression, proliferation and tumorigenicity of human pluripotent stem cells. Nature Communications, 5. https://doi.org/10.1038/ncomms5825
Beroukhim, R., Mermel, C. H., Porter, D., Wei, G., Raychaudhuri, S., Donovan, J., Barretina, J., Boehm, J. S., Dobson, J., Urashima, M., McHenry, K. T., Pinchback, R. M., Ligon, A. H., Cho, Y. J., Haery, L., Greulich, H., Reich, M., Winckler, W., Lawrence, M. S., … Meyerson, M. (2010). The landscape of somatic copy-number alteration across human cancers. Nature, 463(7283), 899–905. https://doi.org/10.1038/nature08822
Diep, C. B., Kleivi, K., Ribeiro, F. R., Teixeira, M. R., Lindgjærde, O. C., & Lothe, R. A. (2006). The order of genetic events associated with colorectal cancer progression inferred from meta‐analysis of copy number changes. Genes, Chromosomes and Cancer, 45(1), 31–41. https://doi.org/10.1002/gcc.20261
Draper, J. S., Smith, K., Gokhale, P., Moore, H. D., Maltby, E., Johnson, J., Meisner, L., Zwaka, T. P., Thomson, J. A., & Andrews, P. W. (2004). Recurrent gain of chromosomes 17q and 12 in cultured human embryonic stem cells. Nature Biotechnology, 22(1), 53–54. https://doi.org/10.1038/nbt922
Garitaonandia, I., Amir, H., Boscolo, F. S., Wambua, G. K., Schultheisz, H. L., Sabatini, K., Morey, R., Waltz, S., Wang, Y. C., Tran, H., Leonardo, T. R., Nazor, K., Slavin, I., Lynch, C., Li, Y., Coleman, R., Romero, I. G., Altun, G., Reynolds, D., … Laurent, L. C. (2015). Increased risk of genetic and epigenetic instability in human embryonic stem cells associated with specific culture conditions. PLoS ONE, 10(2). https://doi.org/10.1371/journal.pone.0118307
Keller, A., & Spits, C. (2021). The impact of acquired genetic abnormalities on the clinical translation of human pluripotent stem cells. In Cells (Vol. 10, Issue 11). MDPI. https://doi.org/10.3390/cells10113246
Kim, Y. J., Go, Y. H., Jeong, H. C., Kwon, E. J., Kim, S. M., Cheong, H. S., Kim, W., Shin, H. D., Lee, H., & Cha, H. J. (2023). TPX2 prompts mitotic survival via the induction of BCL2L1 through YAP1 protein stabilization in human embryonic stem cells. Experimental and Molecular Medicine, 55(1), 32–42. https://doi.org/10.1038/s12276-022-00907-9
Lei, Y., Duong, M. C., Krivec, N., Janssens, C., Regin, M., Huyghebaert, A., Couvreu de Deckersberg, E., Sermon, K., Al Delbany, D., & Spits, C. (2025). Loss of 18q Alters TGFβ Signalling Affecting Anteroposterior Neuroectodermal Fate in Human Embryonic Stem Cells. Cell Proliferation. https://doi.org/10.1111/cpr.13813
Markouli, C., De Deckersberg, E. C., Regin, M., Nguyen, H. T., Zambelli, F., Keller, A., Dziedzicka, D., De Kock, J., Tilleman, L., Van Nieuwerburgh, F., Franceschini, L., Sermon, K., Geens, M., & Spits, C. (2019). Gain of 20q11.21 in Human Pluripotent Stem Cells Impairs TGF-β-Dependent Neuroectodermal Commitment. Stem Cell Reports, 13(1), 163–176. https://doi.org/10.1016/J.STEMCR.2019.05.005
Merkle, F. T., Ghosh, S., Genovese, G., Handsaker, R. E., Kashin, S., Meyer, D., Karczewski, K. J., O’Dushlaine, C., Pato, C., Pato, M., MacArthur, D. G., McCarroll, S. A., & Eggan, K. (2022). Whole-genome analysis of human embryonic stem cells enables rational line selection based on genetic variation. Cell Stem Cell, 29(3), 472-486.e7. https://doi.org/10.1016/j.stem.2022.01.011
Merkle, F. T., Ghosh, S., Kamitaki, N., Mitchell, J., Avior, Y., Mello, C., Kashin, S., Mekhoubad, S., Ilic, D., Charlton, M., Saphier, G., Handsaker, R. E., Genovese, G., Bar, S., Benvenisty, N., McCarroll, S. A., & Eggan, K. (2017). Human pluripotent stem cells recurrently acquire and expand dominant negative P53 mutations. Nature, 545(7653), 229–233. https://doi.org/10.1038/nature22312
Molina-Ruiz, F. J., Introna, C., Bombau, G., Galofre, M., & Canals, J. M. (2022). Standardization of Cell Culture Conditions and Routine Genomic Screening under a Quality Management System Leads to Reduced Genomic Instability in hPSCs. Cells, 11(13). https://doi.org/10.3390/cells11131984
Nguyen, H. T., Geens, M., Mertzanidou, A., Jacobs, K., Heirman, C., Breckpot, K., & Spits, C. (2014). Gain of 20q11.21 in human embryonic stem cells improves cell survival by increased expression of Bcl-xL. Molecular Human Reproduction, 20(2), 168–177. https://doi.org/10.1093/molehr/gat077
Rouhani, F. J., Zou, X., Danecek, P., Badja, C., Amarante, T. D., Koh, G., Wu, Q., Memari, Y., Durbin, R., Martincorena, I., Bassett, A. R., Gaffney, D., & Nik-Zainal, S. (2022). Substantial somatic genomic variation and selection for BCOR mutations in human induced pluripotent stem cells. Nature Genetics, 54(9), 1406–1416. https://doi.org/10.1038/s41588-022-01147-3